Emily Lakdawalla • Oct 21, 2010
LCROSS finds lots of water in accessible places at the Moon's south pole -- but we'll have to tread carefully
It is an "opportunity for the future": an intentional crash landing onto a permanently shadowed crater on the Moon has produced evidence of large deposits of water. And that's not all. The water is accompanied by other volatile materials like ammonia, formaldehyde, and mercury. It is most likely held within a "fluffy" lunar soil that would be easy for future explorers to dig into. And these deposits probably extend beyond the Moon's permanently shadowed spots into "permafrost" deposits in sunlit regions that would be much more benign environments for exploration by robots or humans than the cold, shadowed places. All in all, the Lunar CRater Observation and Sensing Satellite (LCROSS) has both achieved its goal of confirming the long-theorized presence of water at the lunar poles and revealed the polar regions to be enticing prospects for both human exploration and scientific discovery.
By just a month after the impact, the LCROSS mission team was able to confirm that they'd found what they were looking for: water lurked in those permanently shadowed regions on the Moon. But it's taken more than a year for the results from the mission to work their way through peer review and be formally published, and that's what the briefing was about today. Here's my notes from the briefing, and from a conversation I had with Peter Schultz afterward.
David Paige went first, explaining the mapping of the temperatures at the lunar poles using the Diviner instrument on Lunar Reconnaissance Orbiter.
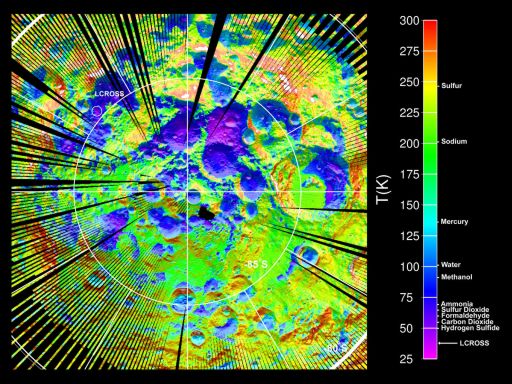
UCLA / NASA-JPL / GSFC
Diviner map of the temperature of the Moon's south pole
LRO Diviner Lunar Radiometer Experiment surface temperature map of the south polar region of the moon. The data were acquired during September and October 2009 when south polar temperatures were close to their annual maximum values. The map shows the locations of several intensely cold impact craters that are potential cold traps for water ice as well as a range of other icy compounds commonly observed in comets. The approximate maximum temperatures at which these compounds would be frozen in place for more than a billion years is shown next to the scale on the right. The LCROSS spacecraft was targeted to impact one of the coldest of these craters, and many of these compounds were observed in the ejecta plume.So, Paige said, roughly 3% of the area near the south pole at the surface is at a temperature below 100 degrees Kelvin. But beyond the shadowed regions, there are some places that receive some direct sunlight during the year that are cold enough to preserve water below the surface. 30% of the area in the south polar region has areas below the surface where water could be stable today, areas that Paige called lunar permafrost.
ext up was Igor Mitrofanov, representing the Lunar Exploration Neutron Detector instrument on Lunar Reconnaissance Orbiter. He said his instrument suggested that the soil within Cabeus' permanently shadowed regions may be up to 4% water by weight. This is important, as Tony Colaprete explained later, because about 1% water by weight is what's currently considered the floor for an abundance that is viable for extraction (not necessarily economically viable, just that if there's less than 1% water you end up spending more energy than you generate by attempting to extract it). More importantly, Mitrofanov argued that the "blue regions" in his data, indicating the presence of relatively large (by which I assume is meant, more than 1%) deposits of hydrogen in the lunar subsurface extend beyond the permanently shadowed areas, into sunlit areas at the poles, corroborating what Paige said. The upper few centimeters of lunar soil appears to be insulating the material below it, preventing the volatiles from warming enough to escape. However, the distribution is lumpy -- the hydrogen is visible in some places but not others. The point is that permanently shadowed regions are no longer required locations for resource utilization, he said. We see hydrogen in illuminated areas. "We may have enough amount of water outside of the permanently shadowed regions, which is very important for future exploration." Permanently shadowed regions are so cold that it would be difficult to operate there; if we can access water from sunlit regions, it would be much, much easier to deal with technologically, for both humans and robots.
Next up was Anthony Colaprete, the LCROSS principal investigator, talking about what they saw in the ejecta plume. The answer: water, more than they had initially thought. All of his visuals were in the form of a video, which I took the liberty of deconstructing. First of all, here are the results of his measurements, indicating that throughout the time that the spectrometer saw the plume, there was an average of 5.9% plus or minus 2.9% water by weight in the material that was ejected from the plume, which is a lot, but is in line with Mitrofanov's observations.
Water mass (kg) | Dust mass (kg) | Total water% | ||
---|---|---|---|---|
Time (sec) | Gas | Ice | ||
0-23 | 83.4 ±25 | 58.5 ±8.2 | 3148 ±787 | 4.5 ±1.4 |
23-30 | 24.5 ±8.1 | 131 ±8.3 | 2434 ±609 | 6.4 ±1.7 |
123-180 | 52.5 ±2.6 | 15.8 ±2.2 | 942.5 ±236 | 7.2 ±1.9 |
Average | 53 ±15 | 68 ±10 | 2175 ±544 | 5.6 ±2.9 |
His presentation also contained this cool series of spectra, which I thought was a really valuable illustration of how spectroscopists read their "squiggly lines" (a term that sounds dismissive, but it's said with affection; it's often used by spectroscopists to describe their own data). You can see how spectroscopists build up a model using a library of spectra of pure materials to determine what materials are present in places out in space.
![]() Fitting a spectrum Five stills from a video released by the LCROSS mission document the work that spectroscopists do to figure out the composition of materials from a remote spacecraft. It begins with the data (black dots), discrete measurements of how much light enters a detector for each small slice of the electromagnetic spectrum. The bars above and below the black dots indicate how uncertain the measurement is; such uncertainty derives both from the behavior of the spectrometer and from how many photons are out there for the spectrometer to detect (the fewer photons, the less certain the measurement). Next, the team attempts to fit the shape of the data using a mixture of models and a library of spectral shapes measured in the laboratory for different materials. First, they model the expected contribution from thermal radiation (red slope at the bottom part of the graph, and the red line at the top of the graph). The black dots are all below what's expected from just thermal radiation, and as a result there's a residual error (blue dotted line). Next, they added in the spectrum from a certain amount of solid water ice (blue line). The model fit improves, especially in the areas of water ice's two main absorptions at wavelengths near 1.5 and 2 microns. Next they added in the contribution expected from the presence of water vapor, which improves the fit near water vapor's absorptions near 1.4 microns and a triplet near 1.8-1.9 microns. A few bumps were left, which require the presence of hydroxyl ions (OH), sulfur dioxide, and methane. The final model fit is a very good approximation of the data, but there is a relatively large "misfit" at 2.2 microns that is not yet accounted for by the model. Credit: NASA / ARC / processed by Emily Lakdawalla |
Next up was Peter Schultz, who explained what the shape of the ejecta plume told us about the surface. The shape and speed of that plume was unusual. Normal impacts send things out instead of up, he said. But this one sent most ejecta straight up, and it was sent up at high speed. There were two reasons: one, the impactor (the Centaur stage of LCROSS' launch rocket) was hollow, and two, the material that the Centaur slammed into was very, very fluffy. I actually called Pete (from whom I took a class on impact cratering a long time ago) to ask him about this fluffiness. Was it fluffier than what the Apollo astronauts walked on? He said yes, much fluffier. He said when you simulate impacts into Apollo-landing-site-like dust, you see an impact flash within nanoseconds. But LCROSS did not see any visible flash of light until 0.3 seconds after the impact. This is a really, really long time and indicates that before material got hot enough to emit that visual flash, it was being compressed. A lot. I joked with him that maybe this material really could swallow astronauts whole, which was a concern about the lunar dust before the first Moon landings, and Pete said that it really might. In fact, this material is so fluffy that it is a lot less like what we usually think of as lunar dust, and much more like the fairy-castle fluffy surfaces we imagine exist on the surfaces of comets.
Returning to the press briefing, Pete mentioned spectral evidence for the presence of hydrogen, hydrogen bonded to other things (C-H bonds and N-H bonds, for instance), sodium, and even silver. One thing that was interesting about his work is that it's all based on the stuff that got lofted into sunlight, high above Cabeus' steep walls. The impact of the Centaur into the lunar surface was actually at low speed as such things go, only about 2.5 kilometers per second; by comparison, the usual speed for the impact of an asteroid onto the Moon is about 20 kilometers per second, and even Deep Impact's crash was 12 kilometers per second. With this relatively slow speed, not a lot of material was vaporized with the heat of impact. It got lofted high above the ground where it eventually came out into sunlight. Within two seconds of the soil grains getting into sunlight, Pete said, the volatile materials were vaporized -- "they were holding on to the grains for dear life, until they got heated up."
Next up was Randy Gladstone, whose Lyman Alpha Mapping Spectrometer found evidence for molecular hydrogen, carbon monoxide, atomic mercury, calcium, and magnesium. There was quite a lot of molecular hydrogen, actually. He said this confirms that the lunar poles are excellent cold traps not just for water but for many other volatiles as well, and he mentioned that things like molecular hydrogen and mercury are useful for resource utilization -- that is, future human explorers.
Rich Vondrak presented more results from Lunar Reconnaissance Orbiter, and talked a bit further about how the crater appeared to be releasing water vapor gradually continuously for the entire four-minute descent of the LCROSS spacecraft following the Centaur impact -- in effect, it was "steaming."
One questioner asked how easy it would be to get water out of the material that LCROSS crashed into. Colaprete answered, demurring a bit, saying that other people had thought much more about this problem than he or the other lunar scientists had. But he pointed out that the fluffy material is much easier to deal with than digging into solidly frozen ground; "you just scoop it up." You can even just warm the surface, he said -- the crater was steaming, and all you need to do is cover an area, then warm it up, to release the water.
However, in my conversation with Pete later, I learned that this ease of accessing the water cuts both ways. I asked Pete what would happen if you stuck a shovel into an area of the type that Mitrofanov was talking about, a place that is not permanently shadowed, where there is ice-bearing material a few centimeters below the surface. Pete said, first of all, that despite all the results shown today we don't really know what things look like much below the surface; all the material that got lofted upward was likely from pretty close to the surface, and the higher things went, the closer it was to the surface when it started. It could be a veneer of material, but we don't know if it's a veneer or not. It's quite possible that it goes very, very deep, and if so, it could be very, very old -- possibly old enough for these deposits to preserve volcanic gases left over from the later stages of the Moon's geologic activity. Secondly, he said, this material is probably so delicate, that even sticking a shovel into the ground might warm it enough to make the water and other, even more volatile stuff (like molecular hydrogen and ammonia) go away -- just the shovel will warm it up.
This fragility makes Pete worry about how these possibly commercially useful deposits of material are going to be approached next. "We don't want Captain Kirk to land there and step out and blow everything away," he said. Even a robotic lander, just by landing, could destroy the materials it was sent to observe. There may be something very ancient held within these deposits of cold materials. He likened it to the thousand-year-old giant sequoias in Redwood National Park -- you wouldn't study them by cutting them down. But just like an old-growth forest, it's a place where commercial interests and the interests of scientists to preserve an ancient and fascinating landscape may not be in alignment. For his part, Pete argued that before commercialization begins, we need to understand it better, otherwise we risk destroying something special. Although I didn't talk to them personally, Colaprete and Mitrofanov seemed much more excited than Pete Schultz was about the possibility of utilizing these deposits as a means to support future exploration, either human or robotic.